For over two decades, high-energy physicists have grappled with a fundamental question about an important quantum property of muons—a class of subatomic particles 207 times heavier than electrons, found in most cosmic rays that reach Earth. Experiments conducted at particle accelerators such as Fermilab in the US and the LHC in Europe have failed to converge with the theoretically predicted value of the muon’s magnetic moment.
This parameter describes how muons wobble (rotate around their own axis) like small magnet bars under a magnetic field. Experiments have found a higher magnetic moment (an anomaly) than predicted or explained by existing theories.
Reconciling this disparity, if indeed genuine and not attributable to methodological errors, could prompt a major overhaul of the so-called Standard Model of particle physics—the foundational theory of physics that has explained interactions between particles and known forces (except gravity) for over half a century.
An article published in December 2023 in Physical Review Letters (PRL), authored by an international group including a Brazilian physicist, has identified the source of the discrepant results produced by two theoretical approaches to estimating the magnetic moment of muons.
“While our paper does not solve the muon magnetic anomaly, it does pinpoint the key factor driving much of the discrepancy between computer modeling and experimental data,” says Diogo Boito from the São Carlos Institute of Physics at the University of São Paulo (IFSC-USP), one of the authors. The underlying factor they found is known as hadronic vacuum polarization, a type of interaction between muons and virtual particles.
In their study, Boito and colleagues from Europe and the US used an approach that isolates the role of this interaction in the emergence of excess magnetic moment, and found it to be a major driver of the observed discrepancy.
“In the past, theoretical work has yielded different values for the contribution of hadronic vacuum polarization to the anomalous magnetic moment of the muon,” comments physicist Farinaldo Queiroz from the Federal University of Rio Grande do Norte (UFRN), who was not involved in the PRL article. “According to the findings from the recent paper, the disparity between experimental and theoretically predicted data may be either more statistically significant than thought or inconsequential.” Simply put, the value of this contribution, the study indicates, determines whether the theoretical calculations truly disagree with measurements taken at accelerators.
The muon’s anomalous magnetic moment is calculated using the formula g-2. The value of the g factor, representing the muon’s magnetic moment, is extremely close to, but slightly greater than, 2. How close it is to 2 defines the ratio of the discrepancy between theory and observation
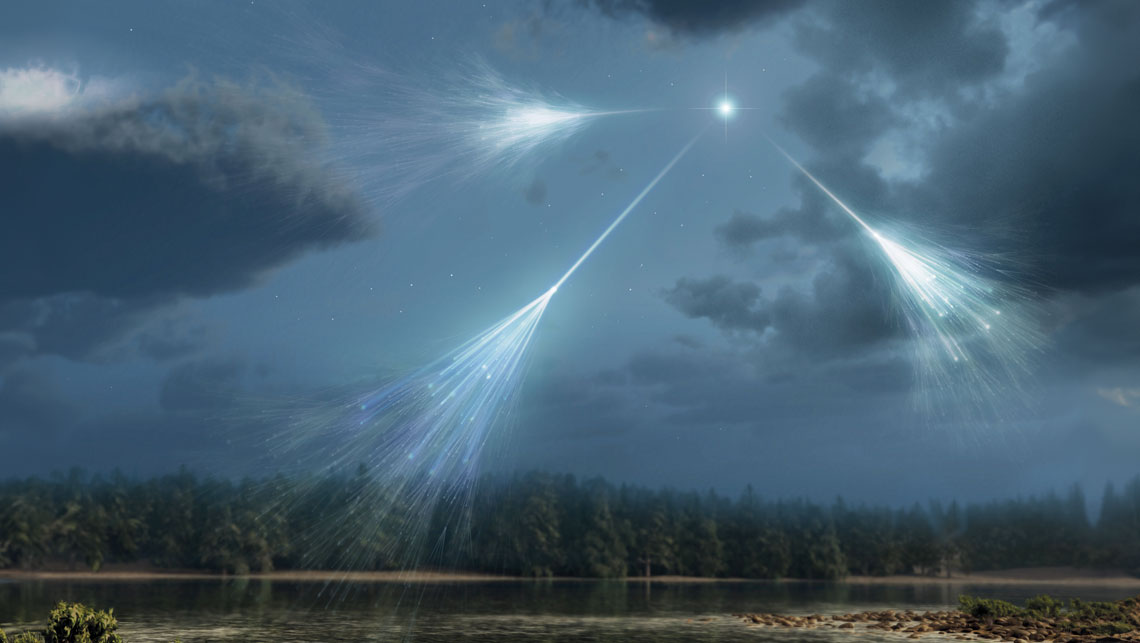
Mark Garlick / Science Photo Library / Getty ImagesIllustration depicting an air shower of cosmic rays, which are largely composed of muonsMark Garlick / Science Photo Library / Getty Images
MUON G-2
In August 2023, the leading experiment dealing with this question, Muon g−2 at Fermilab, published the most precise measurements of this parameter to date. The new value, also published in PRL, doubled the precision of the previous measurement, with a margin of error of 0.20 parts per million (ppm).
It is now up to theorists to demonstrate that their calculations are not flawed. This is where the role of hadronic vacuum polarization—an intricate type of interaction between muons and virtual hadron particles—comes into play. Even more fleeting than the 2.2 millionths-of-a-second half-life of muons, virtual particles are a purely quantum phenomenon. They can be emitted by other particles or arise and vanish almost instantaneously in a vacuum.
“Virtual particles are not directly detected,” explains Boito. But their existence can be inferred as they induce changes in the physical properties of other particles—such as their mass, electric force or, as in the case of muons, their magnetic moment. Hadrons are a class of subatomic particles composed of even smaller particles held together by strong nuclear force. Protons and neutrons are the best-known hadrons, but there are over a hundred of them. Which virtual particle causes the excessive wobbling of muons remains unknown.
According to Queiroz, the new study leaves the door open for the Standard Model to one day be revised and possibly include new particles that help account for phenomena inadequately explained by the theory, such as the muon’s anomalous moment or the nature of dark matter. “When physicists encounter a problem in nature they can’t solve, they propose the existence of a new particle,” quips the UFRN researcher, part serious, part jest. “This is what led physicists to first propose the Higgs boson, for example. In this case, the theoretical particle [which imparts mass to other particles] was ultimately confirmed.” However, the excess magnetic moment of the muon has continued to challenge physicists for decades.
Project
Standard Model Tests: Precision QCD and muon g-2 (nº 21/06756-6) Grant Mechanism Young Investigator Award; Principal Investigator Diogo Boito (USP); Investment R$622,024.29.
Scientific articles
BENTON, G. et al.Data-Driven determination of the light-quark connected component of the intermediate-window contribution to the muon g-2. Physical Review Letters. Vol. 131, no. 25. Dec. 22, 2023.
AGUILLARD, D. P. et al. Measurement of the positive muon anomalous magnetic moment to 20 ppm. Physical Review Letters. Vol. 131, no. 16. Oct. 20, 2023.