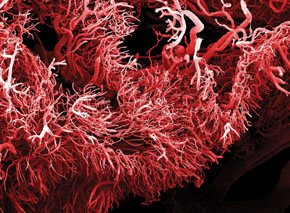
Photo Clouds Hill Imaging Ltd / Science Photo LibraryTangled network: microscopic image of the blood vessels of an intestinal tumorPhoto Clouds Hill Imaging Ltd / Science Photo Library
An international team of biophysicists led by a Brazilian scientist, José Onuchic of Rice University has developed a mathematical model that explains in detail how cells of the walls of blood vessels respond to the nutritional needs of a growing tumor. If the model’s predictions are confirmed, its mathematical equations may help researchers discover more effective compounds to prevent the formation of blood vessels that feed tumors, without interfering with the body’s other blood vessels.
Tumor cells, like other cells of the body, need oxygen- and nutrient- rich blood to survive and multiply. When the cells of healthy tissue require extra blood, they secrete chemicals that stimulate the development of new blood vessels. The principal substance is the vascular endothelial growth factor, or simply VEGF. Upon reaching the wall of the nearest blood vessel, the VEGF molecules stimulate new blood vessels to sprout from existing ones and grow toward the cells that sent the signal.
Growing tumors do something similar. The difference is in the amount of VEGF produced. If the signal sent by normal tissues is, let’s say, a polite request, that of cancerous cells is an urgent request. This stronger signal is an order for the surrounding cells of the blood vessels to quickly multiply towards the tumor. Instead of a harmonious network of blood vessels, with an ordered structure and slow growth, in which small vessels sprout from larger vessels, they form a network of numerous rickety vessels that grow quickly to feed the cancer cells.
“Since cancer cells grow very quickly, they need a great deal of oxygen and to get it they send out a VEGF signal, which promotes chaotic blood vessel formation,” says Brazilian biophysicist Marcelo Boareto, the lead author of the article describing the model, which was published in the July 2015 issue of the Proceedings of the National Academy of Sciences (PNAS). Currently pursuing a postdoctoral internship at the Swiss Federal Institute of Technology (ETH), Boareto developed the model for blood vessel growth in partnership with Mohit Kumar Jolly, of Rice University. In the model, Boareto applied the conclusions of his doctorate, done under the guidance of Onuchic and Eshel Ben-Jacob, a Rice biophysicist and also of the University of Tel Aviv in Israel. “This type of research shows that advances in medical science now often require integrating theory with carrying out experiments,” says Onuchic.
Ben-Jacob, who died in June 2015, pioneered the creation of mathematical models to try to understand how cells—forming a colony of bacteria, a healthy tissue or a tumor—communicate with each other through chemical signals. His models, made in partnership with the biophysicist Herbert Levine, also of Rice, explain why nearly genetically identical cells can behave very differently depending on the signals they receive from neighboring cells.
This chemical conversation between cells is important, for example, during the development of an embryo. “To form a multicellular organism from a cluster of identical cells, the cells need to multiply and differentiate,” says Boareto. “Interestingly, most of the changes occurring in the cells during embryonic development are coordinated by only a few signaling systems.”
One is the Notch system, which is essential to the development and maintenance of various tissues of the human body, including vascular tissue. The system works as follows: the membranes of the cellular walls of blood vessels contain a protein called the Notch protein. Part of this protein gets outside the cell and can connect to two proteins: delta and jagged.
Interspersed or grouped
In an article published in February 2015, Boareto, Jolly, Ben-Jacob and Onuchic for the first time explained, through a system of mathematical equations, how the Notch system acts on the cell differentiation of all tissue. Experiments carried out by other researchers in recent years had already shown that the cells of tissues rich in delta protein, as a result of a signal known as lateral inhibition, arrange themselves like pieces on a chessboard: cells with delta proteins on their surface are interspersed with cells containing Notch proteins. Yet in tissues with plenty of jagged protein, a form of signaling called lateral induction prevails, which leaves very similar neighboring cells with both the jagged and Notch proteins on their surfaces.
In healthy tissue, the supply level is signaled by the VEGF protein, which activates both lateral inhibition and induction. When in balance, these two forms of signaling allow the formation of robust blood vessels. The lateral inhibition leads to the appearance of tip cells, which guide the growth of the vessels, while the lateral induction gives rise to stalk cells, which form the vessel’s wall. “The tip cell is able to move toward the source of VEGF and leads the formation of a new vessel, as the immobile stalk cells multiply and form the wall of the new vessel,” notes Boareto.
Rickety blood vessels
When they decided to test the model, Boareto and his collaborators thought that the accelerated growth of the blood vessels resulted from excessive production of the delta protein, which would favor the appearance of a large number of tip cells. But experiments done in 2007 and 2009 indicated otherwise. By deactivating the gene responsible for the production of delta protein, many blood vessels began to grow, and very close to each other, as happens in the vicinity of a tumor. “The results of the experiment surprised us,” says Boareto.
Contrary to what was once thought, the VEGF signal emitted by tumors, combined with signs such as inflammation, appears to suppress production of the delta protein and increase jagged synthesis, causing the cells that form the wall of the vessels to proliferate.
Jolly and Boareto carefully analyzed the equations of their model so as to understand how to reconcile what they knew about the Notch signaling system with the results of experiments using blood vessels. The solution they found was to assume that the rickety vessels feeding the tumors are unlike normal vessels, which are made up of tip cells and several stalks.
Jolly and Boareto are suggesting that these vessels are made of hybrid cells, with intermediate properties somewhere between tips and stalks: not as mobile as the former, nor able to multiply like the latter (see infographic). “Our hypothesis is that an excess of jagged protein produces hybrid cells, forming many thin, immature and inefficient vessels,” says Boareto. “Cancer seems to take advantage of already existing mechanisms, that healthy cells need to survive, adapting them to its needs,” says Onuchic.
To test this hypothesis and validate the model, Boareto says they need experiments to monitor how the cells of a blood vessel with excess jagged protein respond to VEGF signaling. “It’s an easy hypothesis to test,” he says. “We are discussing this with experimental groups.”
The hypothesis, if confirmed, may lead to a new way of controlling blood vessel formation around tumors. The anti-angiogenesis compounds available today block the proliferation of blood vessels by obstructing the workings of Notch proteins. The problem is that these drugs prevent blood vessel formation not only near the tumor, but also in the rest of the body, which can further weaken the health of cancer patients. The model conceived by Boareto and his colleagues paves the way for a different strategy: using a compound that further amplifies the tumor’s VEGF signal. If it works as expected, this signal would stimulate greater production of jagged proteins and create more blood vessels. The expectation, however, is that these vessels will be exceptionally thin and unable to transport blood to the tumor.
In an e-mail message, the Brazilian researchers Renata Pasqualini and Wadih Arap, at the School of Medicine of the University of New Mexico, noted that “while these predictions are exciting, they still await experimental validation.” The two oversee a laboratory that is an international reference in the search for new treatments for various diseases, by exploring differences between the proteins of healthy and diseased blood vessels (see Pesquisa FAPESP Issue nº 190) For them, the new model may serve as a guide to the development of drugs that act on abnormal blood vessel formation. Such abnormalities are seen not only in tumors, but also in arthritis and diseases associated with retinal problems.
Scientific articles
BOARETO, M. et al. Jagged mediates differences in the normal and tumor angiogenesis by affecting tip-stalk fate decision. PNAS. July 21, 2015.
BOARETO, M. et al. Jagged-Delta asymmetry in Notch signaling can give rise to the Sender/Receiver hybrid phenotype. PNAS. February 3, 2015.