Standing in the control room of the Carnaúba light beamline in the Sirius laboratory in Campinas, molecular scientist Douglas Galante fires up his tablet and announces: “We’re doing new science here. And we’re just getting started.” The tablet screen displays the image of a bacteria shell capable of digesting and excreting metals such as iron and manganese. Discovered on a beach in eastern Canada, this microfossil is 20–50 micra in length (1 micron, plural micra, is a thousandth of a millimeter long).
Preliminary analyses indicate that the organism which once occupied the shell may indeed have lived for some 3.7 billion years as proposed, though not without controversy, by researchers from the UK in March 2017 in the journal Nature. “We’re close to resolving this dispute by demonstrating that the proposed age is in fact correct, because the way the minerals are arranged is typical of live organisms,” says Galante. If this is achieved, records of the oldest signs of life on Earth may be extended by 300 million years.
Galante’s team of geologists and physicians from the University of São Paulo (USP) identified the form and composition of microfossils, also from Canada and described in a 2020 article in Scientific Reports, as being 1.8 billion years old. A July 2022 study published in Frontiers in Earth Science detailed the roulade-like structure, and the chemical composition of the Conophyton cylindricus microorganism carapace, found in sedimentary rocks in Minas Gerais State and aged between 1.2 billion and 900 million years.
Running since December 2021, and opened in 2023 for the first user groups, Carnaúba, 143 meters (m) in length, is the longest of 10 beamlines currently functioning. Similarly to the others, it harnesses energy radiated by electrons accelerated in Sirius’s circular ring, producing synchrotron light, which is used to study different materials (see Pesquisa FAPESP issues 269 and 287). Inaugurated in November 2018, Sirius is part of the Brazilian Synchrotron Light Laboratory (LNLS), which in turn is part of the Brazilian Center for Research in Energy and Materials (CNPEM). A further four beamlines are being assembled and are set to enter into operation in the coming years.
“Carnaúba is the first and only X-ray nanoprobe operating in the southern hemisphere,” says physician Hélio Tolentino, who participated in its design and construction from 2015 after working for 10 years in the French city Grenoble on a similar synchrotron to Sírius—only Sweden has another of the same type. “The greatest challenge is accuracy, because the light beam must be kept steady on the sample, with oscillation of few nanometers [millionths of a millimeter], after travelling 143 m between source and focus to the sample being studied.”
Tolentino compares the beamline to that of an electronic microscope, the former differing in its capacity to penetrate any type of material, even in solution form, to operate in the X-ray range with power levels between 6,000 and 15,000 electron volts (eV). The electronic microscope, in comparison, is limited to the surface of materials, and other X-ray techniques cause destruction of the material studied when searching for information about their interiors.
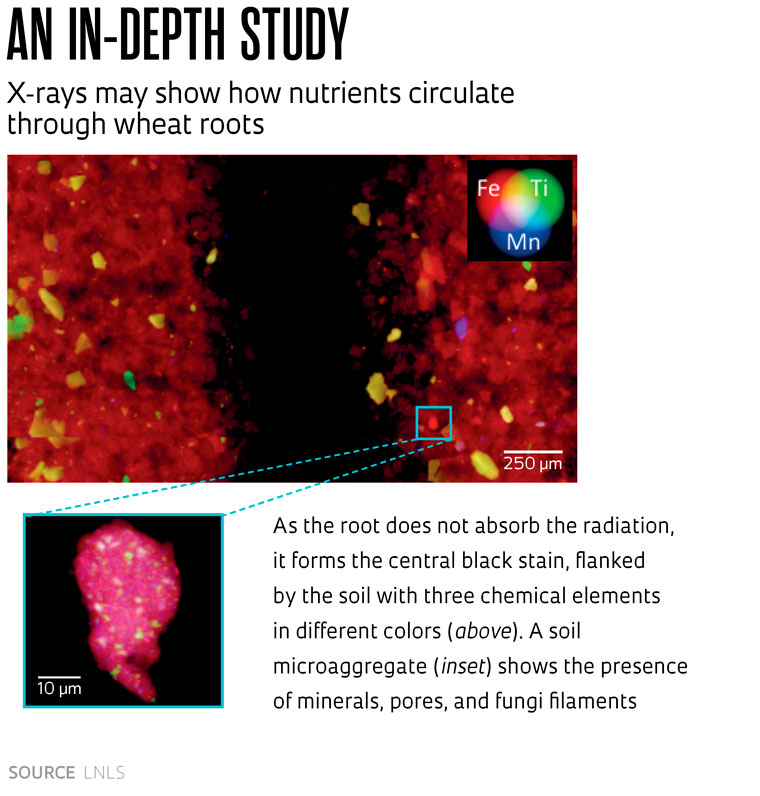
Tolentino lists other advantages of using an X-ray microscope—which works with electromagnetic radiation—over an electronic one based on electron beams: “Greater X-ray penetration into the material, and the possibility of constantly varying the power level and performing spectroscopy [identification of chemical elements using electromagnetic radiation], providing data on the chemical and electronic states of materials.” The relativistic electron beams (which move close to the speed of light) emit concentrated light, whereas in more conventional X-ray apparatus, which reveal shadows on the lungs or broken bones, the radiation takes the form of a wide spectrum.
“We can now study materials to a spatial resolution of a dozen nanometers under different experimental conditions,” he comments, drawing on examples of ongoing studies. “We can examine a solar cell operating in an environmental simulator. It is also possible to study radiation-sensitive samples such as cells and biological tissue, or an electrocatalyst formed from an enzyme in an electrochemical cell.” An article in the Journal of Electron Spectroscopy and Related Phenomena in July demonstrates that the results of studies undertaken in the first two years of the beam’s operation have varied.
Facing the glass walls of the control room, behind which there is a corridor and the experimental station—closed off and shielded during X-ray emissions—there is a 2-meter-high monitor screen showing the image of a plastic vase containing a wheat seedling. Below the monitor, six computer screens display graphics that refresh constantly. “With these monitors we can see everything that happens within the experimental station,” explains Argentine physicist Carlos Pérez, coordinator of the Carnaúba beam, who had been monitoring the experiment to 10:30 p.m. the day before. “We can make adjustments remotely.”
The vase is very small: 3 centimeters (cm) high, by 1 cm in diameter. It’s difficult to find it among all the experimental station wiring and apparatus when, around midday, the test ends and the synchrotron light is blocked; Pérez opens the shielded door, goes in, and points it out.
By applying X-rays to the wheat seedling root, which has infiltrated into a small tube of 1.5 mm in diameter, and with three mm of soil, the LNLS group intends to see how the plant absorbs nutrients, and how to intervene in the process. If successful, the ongoing test will serve to show the root tissue soaking up the calcium released by hydroxyapatite, the mineral used to restore teeth and bones.
To demonstrate their progress to date, Tolentino shows two images on the wall-mounted screen adjacent to the monitor. The first shows a wheat root with a black stain from allowing the X-rays to pass through it, flanked by a mass with red, green, and yellow polygons, corresponding to iron, titanium, and manganese—the colours differ because each chemical element exhibits a different response when submitted to radiation.
The second image is that of a soil microaggregate—a nanoclod—containing organic material and metals. “It’s the first time that we have distinguished metallic chemical soil elements, and we can see the pores, formed by air, that the water, the plant roots, and the fungi filaments pass through,” says Tolentino.
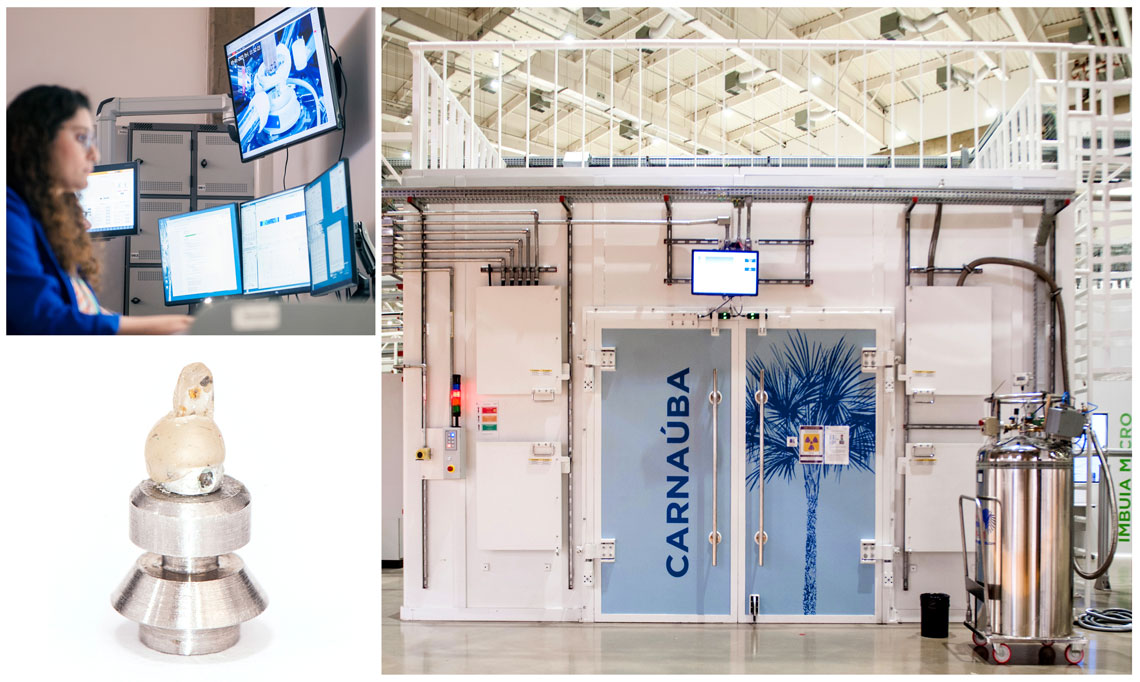
Léo Ramos Chaves / Pesquisa Fapesp
Geologist Carolina Camarda follows experiments from the control room (above left); part of the beamline structure (right); impurities in a diamond demonstrate how minerals behave in the earth’s interiorLéo Ramos Chaves / Pesquisa FapespAmerican soil scientist Dean Hesterberg, of North Carolina State University, participates in the experiments to discover how nutrients, primarily phosphate, retreat into the pores of microagglomerates. He hopes that the data produced will inspire strategies to use less phosphate or repurpose it—global stocks are declining rapidly. “Plants use only 30% of the phosphate, and the other 70% remains in the soil, likely hidden in the micropores,” he says. His hypothesis is that iron oxide, common in Brazilian soils, may attract phosphate and hinder its incorporation by plant roots.
“We haven’t yet been able to detect phosphorus in microagglomerates, because any obstacle or even the air itself weakens the signal it emits,” explains Tolentino. However, he hopes that phosphorus will appear when a more powerful source is installed for low-energy beamlines, forecast for operational start-up in mid-2024.
The work done to date is causing excitement among researchers, and the feeling of entering into a new realm, as occurred with the inventor of the microscope, Dutch naturalist Antony van Leeuwenhoek (1632–1723), when he observed plants and cells in detail for the first time. Issues can also arise, however, because the 3D images produced from the measurements at the experimental station often do not match with anything known. “We are learning to interpret these images, which have never been seen before,” comments Tolentino.
Unlike Leeuwenhoek and his microscope, they have to convince the other scientists that the image is real and carries scientific logic. Geologist Carolina Camarda, of the University of Brasília (UnB), did just that on using several Sirius beamlines to confirm her conclusions.
Camarda studies impurities in so-called superdeep diamonds, formed more than 200 kilometers (km) down in the earth’s mantle. These very rare stones maintain their original structure, and may therefore indicate how minerals behave under high pressures and temperatures deep inside the earth. Geologists Tiago Jalowitzki and Fernanda Gervasoni were given 10 superdeep diamonds, each 2–3 mm in diameter, from a cooperative of artisanal miners in the Juína region of Mato Grosso State, at its border with the state of Rondônia, one of the few places in the world where they are found.
In one of the samples, known as J1, analyses revealed two iron ores—haematite and goethite, both very different from the form in which they are found on the earth’s surface. “As both are formed under a pressure of between 54 and 60 gigapascals [GPa], close to the breakdown limit for these materials, the distance between the atoms and the deformation is much greater,” he reports. Tolentino adds: “The crystals are imperfect; the same structure does not repeat.”
The images, made up of hundreds of measurements, also reveal sulphides formed of chalcopyrite, pertlandite, and pyrrhotite, common in diamonds. Once again, however, there are differences. Compared with those found on the surface, the deep crystals “are deformed,” observes Tolentino.
Other thought-provoking results are set to be produced by the computers digesting information generated by X-ray emissions, perhaps at an even faster pace. A further experimental station is set to be built next year in Carnaúba, because one alone cannot keep up with the demand, even operating 24/7. “The agenda is full until December 22,” says Tolentino.
Start-up develops compact X-ray equipment
In the hangar of a business park close to the Dom Pedro I Highway in Campinas, São Paulo, the Pitec development team tests the parts of a compact X-ray detector. “We hope to have the first prototype ready by the end of this year, and launch the finished version during the first quarter of 2024 for scientific experiments and material analyses,” says computer scientist Paulo Bertolo, director of operations.
Provisionally known as RAD, the apparatus is an initiative by the company to conquer new markets. Since 2017, when it started operations in a 25-square-meter room at the University of Campinas (UNICAMP), the company manufactured bigger X-ray detectors, with up to 144 X-ray sensors (compact versions may have fewer than 10), which produce 2,000 images per second with resolution of up to 9 million pixels. Normally painted lilac, the detectors were installed in 6 of the 10 beamlines then operating at Sirius and tested in synchrotron light accelerators from other countries.
The company was born of a meeting at UNICAMP with researchers at Sirius, then under construction. Upon hearing that Sirius needed Brazilian companies to assemble electronic circuits from components imported from Europe, Bertolo and the other partners readied themselves to do so. They did the work and delivered the goods, and then asked if there were any other challenges they could solve. They were tasked, in partnership with LNLS engineers and physicists, to prepare a Brazilian version of a complex X-ray detector, whose delivery lead times are lengthy, and maintenance expensive. Those used at the time came from Switzerland.
Bertolo and other Pitec colleagues, who had formerly worked at the Telecommunications Research and Development Center (CPqD), finished the first prototype in 2019, commencing delivery the following year. To date they have delivered 12 items to LNLS—each can take up to 10 months to manufacture, but this is a shorter period than those imported from overseas. The detectors function in a similar way to a photographic camera, forming images with radiation spread by the objects examined.
In 2020 Pitec was acquired by a business group from Campinas, and then in 2022 by Lumentum, a US-based telecommunications multinational. “Our challenge now is to refine the equipment, offer detectors to the 50 synchrotron accelerators in other countries, and apply the technology across other markets,” says Bertolo.