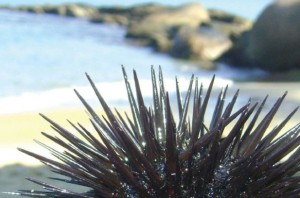
JULIANA SCIANI / BUTANTAN INSTITUTESea urchin: after being pricked, an anti-inflammatory drug can be usefulJULIANA SCIANI / BUTANTAN INSTITUTE
A single type of toad, Rhinela granulosa, produces different venoms depending on whether it lives in the caatinga scrubland or in woods such as the Mata Atlantica (Atlantic rainforest). A jararaca, i.e., the Bothrops atrox pit viper from the Amazon region, produces different venom in the state of Maranhão and in the northwest of the state of Amazonas. In the same place, male and female Bothrops atrox inject venoms of different components into their prey. The composition and therefore the lethalness of the venoms may vary in the same animal: the Siphonops annulatus, a species of amphibian, makes different toxins in its head and its tail, which is where it is most often attacked when it buries itself or enters holes. As for bees, they make venom with a honey-like smell and with ingredients that vary depending on the temperature and the season of the year.
These combinations of toxins, which were previously hardly differentiated, are now acquiring their own identities, as their ingredients and the likely biological function of each become better known in the laboratories of the Butantan institute, the main reference center for the production of serum against venomous animals.
It is now clear that the efficacy of the treatment can increase as one adds information about the origin, environment, age and diet of the venomous animal. Thus, the serum against the bite of a jararaca pit viper bit from São Paulo may fail to placate completely the effects of a bite of a specimen from the north of Brazil. The Bothrops snakes account for about 80% of the 20 thousand accidents with serpents recorded in Brazil per year, with a mortality of 10% among people not treated with serum. The cascavel rattlesnakes, on the other hand, account for 10% of the cases, but with a mortality of 75%.
The increasing knowledge about venom components may even become useful to treat the victims of accidents with less dangerous animals, such as Echinometra lucunter sea urchin, a frequent source of seashore accidents. “The venom of this urchin doesn’t kill, but it’s worthy of respect,” says Daniel Carvalho Pimenta, a researcher at Butantan. Under his guidance, Juliana Sciani analyzed the venom released by a sea urchin’s spine and found that the swelling (or granuloma) at the pricked site causes severe inflammation that can last for days, although it generally does not draw much attention. Therefore, says Daniel, “prescribing an anti-inflammatory drug and an analgesic can help a lot after the accident.”
For the animals, the venoms express their strategy of survival. “The poison makes the life of the venomous snakes easier, allowing them to be thin, whereas boa constrictors, which are not venomous, have to work harder to feed themselves: they kill by wrapping themselves around their prey and suffocating it,” notes Carlos Jared, a Butantan biologist who has been researching, for two decades, the origin and likely functions of these combinations of toxins that represent the continuity of the life of some and the end of the life (or at least a lot of pain) of others.
Kill or merely frighten
Jared is well aware of what a snake can do: he has been bitten three times, once by a cascavel rattlesnake and twice by jararaca pit vipers. Luckily, he was at Butantan itself and prompt medical care was at hand. In 1984, he was demonstrating to a group of visitors how a pair of newly imported boots could shield one from cascavel bites. He stretched his leg and the cascavel attacked, but the boot did not provide the expected barrier, as everyone had assumed. “I felt the teeth of the cascavel injecting the poison in my leg. I have always said that one has to keep calm on such occasions, but I was unable to. I´ve never run to hospital so fast.”
Poison is a means of defense that many animals use to hunt or avoid being hunted. Whereas snakes, scorpions and spiders attack at the slightest sign of danger or of food in the vicinity, amphibians, such as toads and frogs, prefer to scare off rather than kill, adopting what Jared calls pedagogical venom. Jared and his team have observed that the Rhinella, in the presence of potential predator, fill their lungs up with air, puff out their body and get their parotoid glands ready to spray venom. Upon biting, these toads, wild animals or domesticated dogs tighten their glands, which then release a toxic milky liquid directly into the predator’s mouth, causing tachycardia and vomiting. A green frog of the Phyllomedusa group has a more refined tactic: it allows itself to be eaten and once swallowed, it releases vomit-inducing substances, so that in less than a minute, it leaps out, whereas the predator remains dizzy and very hungry.
Sometimes the venom depends on the diet. The dendrobates (a type of frog with blue, green or yellow skin, at most three centimeters long, living mainly in the Amazon region) feed on ants, beetles or acari that, in turn, feed on poisonous fungi. The dendrobates sequester this poison, which accumulates in their skin glands. That is how one dedrobate species, Phyllobates terribilis, incorporates the powerful batrachotoxin, produced by beetles and perhaps by other insects as well. Jared compared the lethalness of the venoms of the Phyllobates and of the jararaca pit viper and concluded that the frog’s poison is 8,850 times more lethal. A bird from Papua New Guinea, the Pitohui dichrous, also eats these beetles and releases this toxin through its skin and feathers. If the feed changes, the venom disappears. The dendrobates at the Butantan vivariums, fed on small cockroaches, are innocuous. “Actually,” says Jared, “they used to be venomous, but they are not poisonous at present.”
This is not always the case. Pimenta analyzed the venom of the five groups of sister-species of the Rhinella living in the caatinga scrubland, in the Amazon region, and in captivity. Externally, they are all yellowish, with a consistency reminiscent of latex from rubber trees, and they dry quickly. The general composition was the same. On the other hand, the Apis mellifera bees, probably in response to temperature variations, produce venoms with different compositions in winter and in summer, causing various types of allergic reactions. In winter, their venom contains one variant (or isoform) of the predominant antigen, melittin, in larger amounts than are found in the main summer venom components. “The experimental serums against the Apis stings only work sometimes, perhaps because they don’t take these variations into account,” says Pimenta.
From viscid to diaphanous
The composition of the venom of two representatives of the same species of jararaca, one from Maranhão state and the other from Amazonas state, can also vary and lead to the production of different groups of antibodies, according to a study by Maria de Fátima Furtado and other researchers from the Butantan Institute. Why are there venom variations among beings of the same species that live in different places? “This might be a sign that the animal is changing and that another species is forming,” comments Jared. “Thousands of years hence, they might be totally distinct species.”
Pimenta finds that venoms evolve from rudimentary combinations such as the yellowish and insoluble goo of an amphibian such as the Siphonops annulatus, to transparent and crystal-clear liquids such as the venom of frogs, spiders and scorpions. “The venom of more primitive animals consists mainly of proteins, whose production requires a lot of energy, and alkaloids, molecules that are far smaller than the proteins,” he says. This is the case of the jararaca’s viscous yellow venom, because of its high protein content. “At the other end, we have venoms with a lot of peptides, with a lower energy cost, and steroids, the chemical structure of which permits may variations,” he adds, exemplifying this with the venom of scorpions, a colorless, fluid consisting principally of peptides.
There are still no clear rules about the chemical variability of venoms within the same species. The cascavel rattlesnake poison varies little and contains about 10 basic components, mainly enzymes that can paralyze other animals’ muscles and nervous system in seconds, according to a study by Airton Lourenço Jr., from Paulista State University (Unesp), based on 112 samples, taken from young and adult serpents from different parts of the country or held in captivity.
However, Pimenta found that the jararaca venom is a mixture of hundreds of components whose proportion varies. Still, it consists mainly of peptides and enzymes that corrode the skin and the conjunctive tissue of the prey, starting to digest them. “The composition of the venoms can be redundant, with a lot of molecules having the same function, to offset the variations in the defense mechanisms of the pray,” he says. Sometimes, molecules with no clear biological function appear. They might be left over from past times, according to Osvaldo Augusto Sant’Anna, a researcher at Butantan and the coordinator of the National Institute of Toxin Science and Technology. “Venom components with no biological function today may have been essential for survival of a species, and may have been kept during the course of evolution; one day, they might become necessary again,” he says. “Just as we acknowledge the diversity among Homo sapiens, identifying individuals through their own characteristics, we must acknowledge that there is diversity within the same species of Bothrops.”
The defense mechanisms that allow birds, the snake themselves, and certain mammals to withstand normally lethal venoms are still uncertain. The Xenodon merremii snake, which is aggressive though non-venomous, has specialized in eating corpulent poisonous toads of the Rhinella genus (previously called Bufo). One type of Brazilian skunk, the Didelphis aurita, from the Mata Atlantica, rainforest, is entirely immune to the poison of the jararaca and partially immune to that of the cascavel. When they are not immune, the animals behave differently. The Nasua nasua coatis scalp the toads, by grating them against stones until the skin with its venomous glands is removed. They then eat the carcass only, and they do so even when they are living in vivariums, far away from the forest.
Scientific Articles
JARED, C. et al. Parotoid macroglands in toad (Rhinella jimi): their structure and functioning in passive defense. Toxicon, v. 54, p. 197-207. 2009.
FURTADO, M. F. et al. Antigenic cross-reactivity and immunogenicity of Bothrops venoms from snakes of the Amazon region. Toxicon. v. 55, p. 881-7. 2010.
FERREIRA Jr., R. S. et al. Africanized honey bee (Apis mellifera) venom profiling: Seasonal variation of melittin and phospholipase A2 levels. Toxicon. v. 56, p. 355-62. 2010.