
Ormuzd Alves / Folhapress
Avenida 23 de Maio in São Paulo, during the blackout that affected 10 Brazilian states on March 11, 1999Ormuzd Alves / FolhapressThe childhood of physicist Yang Yang was marked by blackouts. She grew up in Beijing at the end of the 1990s, when the capital of China suffered from frequent blackouts. “The power plants could not meet the high demand for electricity in the summer,” says the Chinese researcher. Last year Yang completed her doctorate in theoretical physics under the guidance of the Brazilian Adilson Motter, at Northwestern University in Evanston, Illinois. She has developed mathematical models that may help reduce the risk of blackouts in the electrical systems of large countries like China, the United States, and Brazil.
Using these models, Yang, Motter, and a colleague at Northwestern, the Japanese mathematician Takashi Nishikawa, identified an algorithm (a sequence of procedures) that makes it possible to recognize the areas in an electrical network that are more likely to fail in succession, causing a cascade effect that can leave states or even an entire country in the dark. This algorithm was described in an article published in January in the journal Physical Review Letters.
Using computer simulations, the researchers tested the algorithm against the Texas electrical distribution grid, one of the largest in the United States. Not only did they successfully reproduce the blackouts that occurred between 2010 and 2013, they estimated the risk that the Texas network could suffer blackouts of different proportions. “These are estimates that engineers can test and use to direct interventions designed to prevent blackouts in the network,” says Motter.
The algorithm was developed as part of a larger research project coordinated by Motter and Nishikawa and funded with US$3.2 million from the United States Department of Energy. In this project, the physicists collaborate with electrical engineers and other professionals from research institutions and energy companies to create control systems that meet the new reality of the U.S. electric sector.
“The Brazilian network is centralized, with a few large hydroelectric plants,” says Motter. “The United States network functions with thousands of plants scattered throughout the country, mostly thermal and nuclear, and it is increasingly adding more renewable energy sources, mainly solar and wind power.” According to the physicist, the problem with these two alternative sources is that they produce energy intermittently, since solar panels do not generate electricity at night or when the sun is behind clouds, and wind turbines do not operate when the wind is still. This intermittent nature, says Motter, increases the likelihood that faults in the energy grid will be amplified and lead to blackouts.

NOAA
Satellite images show city lights along the northeastern seaboard of the United States in white, on August 13, 2003…NOAANo matter how well equipped or planned, no power generation and distribution network is free from the risk of a major blackout. The danger is greatest in summer, when heat increases the demand for energy to power air conditioners. At the same time, climatic extremes like droughts and storms, typical of the season, increase the likelihood of fires and lightning, which can shut down power generators and disconnect power lines.
Blackouts are more than just an inconvenience: they cause serious economic damage. It is estimated that the United States loses tens of billions of dollars per year from stoppage of industrial activities and services as a result of blackouts, and Brazil faces the same problem. A survey by the Brazilian Center for Infrastructure (CBIE) concluded that there were 181 blackouts in several regions of the country between 2011 and 2014.
“Major blackouts are rare, but small blackouts happen all the time,” says Motter, who earned a degree in physics from the University of Campinas (UNICAMP). Since 2006 he has worked at Northwestern as a specialist in complex network dynamics, an area of mathematics that studies the function of systems formed by many interconnected components, like networks of neurons in the brain or those formed by power plants, transmission lines and electricity distribution systems. In such complex systems, a failure in one component causes others to fail in succession.
“When one transmission line is interrupted, parallel lines can become overloaded and shut down automatically to prevent permanent damage to the system,” states Motter. Some of these cascades of failures cause blackouts in small parts of the network, while others take on large proportions. “We are trying to understand how these cascades propagate and what determines their size,” adds the physicist.
In the United States, the largest and most recent national blackout took place on August 14, 2003, and affected large portions of the Northeast and Midwest, as well as the province of Ontario in Canada. It began with the initial failure of three transmission lines in Ohio, and in less than an hour 255 power plants were offline. At first, the plants stopped gradually, but in the last two minutes of this hour-long period, most of them shut down almost simultaneously.
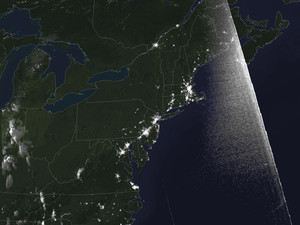
NOAA
…and during the blackout on August 14NOAAOne of the largest blackouts in Brazil took place on November 10, 2009, and began with a failure in a substation in Itaberá, in the countryside of São Paulo State. The problem triggered a cascade that led to the shutdown of the Itaipu hydroelectric plant and affected entire states in the Southeast and Central-West of the country, as well as Paraguay. “There was an amplification effect,” said Motter. “When Itaipu, which produced 20% of the country’s energy, went offline, it induced other parts of the network that were indirectly connected to the plant to also go offline, and the blackout affected approximately 40% of national demand.”
Backbone
It is difficult to estimate the probability that major blackouts like these will occur, since they are relatively rare. The historical data of an electrical network are not sufficient for physicists to apply statistical analysis to extreme events. In their study of the Texas electrical network, Motter and his colleagues tried to circumvent the problem by using computers to create as realistic a model of the network as possible.
Each simulation began by replicating a real-life energy supply and demand situation, as well as the transmission capacity which the power lines in Texas experienced at peak times between 2010 and 2013. From these real situations, the researchers simulated imaginary blackouts by triggering random failures in some elements of the virtual network. Afterwards, they recorded the size of the area affected by the cascade induced by these failures.
The data from 120,000 virtual blackouts revealed something surprising: the physicists noticed that often the failure of one element in a network may spare its neighbors and induce failure in other, more distant elements. Groups of dozens of elements without direct connections tended to fail together in a large blackout. The researchers also found that the size of the blackouts depended more on the connections between the elements of a group and the size of this group, rather than the size and connections of the electrical network as a whole.
“Several factors could affect the size of failure cascades,” says Motter. “Our work has identified two determinants.” One is the probability that each element of the network will fail alone. The other is the risk that two or more elements will fail together in the same cascade.

Takashi Nishikawa / Northwestern University
Map of the US electrical grid shows the most connected pointsTakashi Nishikawa / Northwestern UniversityIdentifying these two factors allowed the researchers to create a simpler model to predict blackouts in the Texas network than those obtained from the realistic simulation which was initially used. “These subgroups of more vulnerable elements form the backbone of the cascades,” says engineer Elbert Macau from the Brazilian National Institute for Space Research (INPE) in São José dos Campos, São Paulo. He leads a research project on complex networks funded by FAPESP, and recently published a study on the blackout problem. “Motter’s group found a very creative method to simplify the calculations required to estimate the probability that a cascade of possible failures will occur in a complex network,” says Macau. “Without this simplification, it would be unfeasible to calculate this for a national electrical network.”
While Motter’s team modeled how a cascade of failures propagates across the electrical network, Macau and his colleagues have tried to get a better understanding of one of the main causes of these failures: the lack of synchrony between energy supply and demand. In November 2016, the INPE group, along with researchers from the Technological Institute of Aeronautics (ITA) and the Federal University of Fronteira Sul (UFFS), published an article in the journal Chaos in which they introduced a mathematical model that focuses on this issue. Using this model, researchers can determine the conditions of stable equilibrium in which an electrical distribution system can operate to maintain synchrony between power generation and consumer demand even when disturbances are present, thus reducing the risk of blackouts. The model was applied to the Brazilian electrical distribution network to determine its tolerance to specific disruptions.
Another project that included Brazilians went a step further, proposing ways to quickly restore synchrony in electrical networks. In another article published two months previously in the same journal, the Chinese doctoral student Chengwei Wang and the Brazilian physicists Celso Grebogi and Murilo Baptista (all at the University of Aberdeen in Scotland) showed that the application of electrical disturbances, calculated from partial information about energy consumption and generation, can prevent major desychronizations from occurring and leading to blackouts. “We have created a reliable system in which there is a permanent balance between generation and demand,” says Grebogi. “The proposed control permits the construction of intelligent electrical networks, smart grids.”
The electrical engineer Antonio Padilha Feltrin, from the Ilha Solteira campus of São Paulo State University (UNESP), finds Motter’s proposed model for identifying groups of network elements that tend to fail together an interesting novelty, but notes: “Obtaining the initial data to calculate the odds is very complicated for the electrical industry.” Feltrin explains that the network is constantly being altered through improvements and maintenance, which can drastically change the likelihood that an element of the network will fail from one moment to the next.
For example, he recalls that after the 1999 blackout in Brazil, a new communication system was established between the country’s main power plants. “The electrical network has changed so that a failure like the one that happened that year can no longer happen the same way,” he says. Even so, according to Feltrin the model made by Motter’s group has potential to help prevent blackouts if the network has a responsive and efficient real-time information system, the main characteristic of the new generation of electrical networks that are being created in the United States: the smart grids.
Scientific articles
YANG, Y., NISHIKAWA, T. and MOTTER, A. E. Vulnerability and co-susceptibility determine the size of network cascades. Physical Review Letters. Jan. 27, 2017.
GRZYBOWSKI, J. M. V., MACAU, E. E. N. and YONEYAMA, T. On synchronization in power-grids modelled as networks of second-order Kuramoto oscillators. Chaos. Nov. 2016.
WANG, C., GREBOGI, C. and BAPTISTA, M. S. Control and prediction for blackouts caused by frequency collapse in smart grids. Chaos. Sep. 2016.