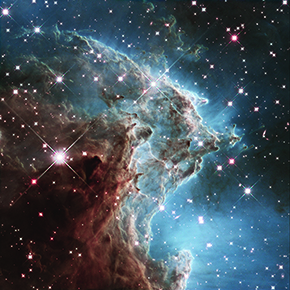
Image NASA, ESA, and the Hubble Heritage Team (STSCI/AURA)
Monkey Head (NGC 2174): image of the nebula taken by the Hubble telescope, containing young stars (bright spots), plasma clouds and molecular clouds (dark regions) (Click for more details)Image NASA, ESA, and the Hubble Heritage Team (STSCI/AURA)It is hard to see the details in the darkest areas of the image of the Monkey Head nebula (NGC 2174), 6,400 light-years from Earth. Overshadowed by the brilliance of the young stars and glowing gas plumes, these dark areas are home to some of the many star-forming regions in our galaxy. It is in precisely these pockets obscured by dust in the interstellar medium that many astronomers are interested. It is there, in what are called molecular clouds, that the gas of the nebula cools enough to trigger a gestation process that, a few million years later, will give birth to new stars.
Today’s telescopes are just beginning to have enough resolution to glimpse the details of this process, which involves a turbulent tug of war between gravitational and magnetic forces. On one hand, gravity tends to make the gas denser, which allows the atoms in the center of the densest regions of the cloud to be compressed until they release energy through nuclear fusion reactions, sparking new stars. On the other hand, the magnetic fields of the cloud tend to push in the direction opposite that of gravity. Everyone knows that gravity wins in the end, but nobody really understands the details of the conflict.
To solve this riddle, a team led by astrophysicist Elisabete de Gouveia Dal Pino, of the University of São Paulo, and by the astrophysicist Alexander Lazarian, of the University of Wisconsin, Madison, have been carrying out simulations of the behavior of the gas and magnetic fields in molecular clouds. The results of their most recent simulations resolve contradictions between theory and observations, lending support to a new idea regarding how magnetic fields hinder, but do not quite prevent, the emergence of new stars. This same idea can explain why the discs of gas and dust around new stars—and that may later lead to a system of planets—do not disappear despite the forces of magnetic fields.
Molecular clouds are very small regions and specific to the interstellar medium that permeates galaxies. These clouds are pockets of gas and dust much colder than the surrounding interstellar medium. While the temperature in the clouds of interstellar gas reaches thousands of degrees Celsius, keeping electrons separate from atomic nuclei and forming a plasma (an electrically charged gas), in molecular clouds the average temperature is around -173 degrees Celsius. For this reason, molecular clouds are mostly composed of neutral atoms that are combined to form molecules—hence the name molecular. But even molecular clouds harbor a small amount of plasma, enough so that the lines of the nebula’s magnetic field are connected to the motion of the gas.
“The plasma there flows in a very turbulent manner,” says astrophysicist Reinaldo Santos de Lima, who completed his PhD at USP under the supervision of Dal Pino and is currently working on part of his post-doctorate research at the University of Wisconsin. He explains that the galaxy’s plasma is constantly stoked by shock waves from explosions of massive stars that are ending their lives, the supernovas. One consequence of this turbulence is that the movement of electrical charges in the plasma amplifies the very weak magnetic fields that permeate space, originating in the early Universe. These more intense fields, up to 0.003 gauss (one hundred times smaller than that on the Earth’s surface), end up affecting the molecular clouds.
Magnetic Necklaces
To explain the phenomenon in her classes, Dal Pino uses an analogy. The plasma particles are linked to the magnetic field lines like beads on a necklace are attached to its strand. “If the gas moves to one side, it drags the field lines with it,” says Lima. “This makes star formation difficult.”
As a denser region—a core—within the cloud contracts due to the gravitational attraction of its own mass, this contraction compresses the magnetic field lines. The result is an hourglass-shaped field (see figure) which exerts a repulsive force against gravity. This force, however, acts only in the direction perpendicular to the magnetic field lines. Thus, the mass continues to accumulate at the center of the core, along the lines, until the gravitational force overcomes the magnetic force and the star is formed.
It was believed that a young star inherits the magnetic field from the core from which it originated. However, when observing newly formed stars, astronomers found the magnetic field measured on their surfaces to be 10,000 times smaller than expected.
This discrepancy between theory and observations suggests that some unknown phenomenon disconnects the magnetic field lines from the movement of the gas during its contraction. This would mean that the lines are not as compressed at the center of the core and so the resulting star has a smaller magnetic field. Astrophysicists’ favorite candidate to explain the less intense magnetic fields was, until recently, a phenomenon known as ambipolar diffusion. “As the cloud collapses, the plasma loses heat through radiation and its electrons recombine with its atomic nuclei,” explains Lima. “This neutral material continues to collapse without dragging the field lines.”
Studies have been confirming, though, that for ambipolar diffusion to be able to expel the magnetic field lines from the center of the core efficiently, the size of the dust grains formed in molecular clouds would need to be different than that estimated. The phenomenon also fails to explain the distribution of the magnetic fields measured by astronomers.
In 2005, Lazarian proposed an alternative to ambipolar diffusion, which had the advantage of not depending on the size of the grains in molecular clouds. His idea is based on the phenomenon of magnetic reconnection. This occurs when two sections of plasma, each carrying magnetic field lines in parallel senses but opposite directions, are forced to collide against one another. In a turbulent plasma, this process occurs very quickly, as demonstrated by Lazarian and Ethan Vishniac in 1999. During the explosive collision, the field lines are cut and pasted into a new configuration, a new sense and direction.
Magnetic reconnection occurs quite frequently and violently on the Sun’s surface, ejecting solar mass into space during magnetic storms. Reconnection explains other astrophysical processes, such as the acceleration of ultra-energetic cosmic rays (see Pesquisa FAPESP Issue nº 200). It was thought, however, that it occurred rarely in molecular clouds. Lazarian, however, proposed that the turbulence of the gas in the clouds could accelerate the process.
Small turbulent eddies could create the conditions needed for magnetic reconnection to occur throughout the cloud. In 2009, Lazarian and astrophysicist Grzegorz Kowal, currently at USP, performed computer simulations showing that magnetic reconnection induced by turbulence really worked. Using Dal Pino’s analogy, the turbulence of the gas could be able to cut and reconnect the strands of the necklaces, releasing the beads and pushing excess strands away from the center of the molecular cloud core.
When he spent a few months in Wisconsin in 2009, working with Lazarian, Lima used the computer code written by Kowal to create a simplified model of the collapse of a core in a molecular cloud. The simulations, published in TheAstrophysical Journal in 2010, indicated that the turbulence of the gas kept the magnetic field lines away from the core, allowing it to collapse due to gravity.
Another of Dal Pino’s PhD students, Márcia Leão, currently a post-doctoral researcher at Unicamp, carried out even more realistic simulations of the process. In an article published in November, 2013 in The Astrophysical Journal, the researchers compared the results of these simulations with observations of cores in molecular clouds performed by astronomer Richard Crutcher, of the University of Illinois at Urbana-Champaign. Leão’s simulations could explain the distributions of gas density and its magnetic field measured by Crutcher better than would be expected by the theory of ambipolar diffusion.
Images: 1 HST 2 Santos-Lima, R. et al MNRAS 2013Maintaining the spin
Millions of years after the beginning of the collapse, the gas and dust from the core of the molecular cloud, which once occupied a volume a few light-years in diameter, ended up concentrated in an area roughly the size of the solar system, with a diameter of hundreds of light-minutes, forming a star surrounded by an accretion disc, which can generate a planetary system (see figure). However, calculations suggest that the magnetic field of molecular clouds prevents the formation of these discs. The field lines slow the rotation of the material in the disc, which eventually falls into a protostar.
Simulations published in 2012 and 2013 by Dal Pino’s team suggest that this problem is also solved by magnetic reconnection induced by turbulence, which transports the excess magnetic lines out of the disc. Later this year, the team hopes to publish a study with Gustavo Guerrero, of the Federal University of Minas Gerais (UFMG), with more precise values related to this magnetic transport, in order to better compare this explanation with other alternatives.
“The theoretical predictions are more advanced than the observations,” comments astronomer Gabriel Franco, of UFMG, who works with data on the magnetic field of a molecular cloud obtained using an instrument mounted on the APEX telescope in Chile. “To give you an idea, the hourglass-shaped field, predicted decades ago, was first observed in 2006.”
The APEX’s antenna was the prototype for the 66 antennas of the Alma observatory, inaugurated in 2013, which will also have an instrument capable of measuring the magnetic fields of cores and protostars. But, to observe all the details, Dal Pino is waiting for another network of radio telescopes now under construction in Argentina, called Llama. She says “We might be able to test our theories with extremely high-resolution observations using interferometry, combining antennas from Alma and Llama”.
Project
Investigation of high energy and plasma astrophysics phenomena: theory, observation, and numerical simulations (nº 2006/50654-3); Grant Mechanism Thematic project; Investigator Elisabete Maria de Gouveia Dal Pino; Investment R$366,341.75 (FAPESP).
Scientific articles
LEÃO, M.R.M. et al. The collapse of turbulent cores and reconnection diffusion. The Astrophysical Journal. v. 777, n.1. Nov. 2013.
SANTOS-LIMA, R. et al. Disc formation in turbulent cloud cores: is magnetic flux loss necessary to stop the magnetic braking catastrophe or not? Monthly Notices of the Royal Astronomical Society. Mar. 2013.