The main symptoms of malaria—fever, fatigue, aches and pain—usually appear 10 to 15 days after the parasite that causes the disease, a protozoan of the genus Plasmodium, is injected into the body by the bite of an infected female Anopheles mosquito. Children, pregnant women, people with HIV, and people who have never had contact with the pathogen before are at higher risk of developing the most serious form of the disease, which causes 600,000 deaths a year worldwide—almost all in Africa (95%). A study led by Brazilian researchers, published in the journal Cell Metabolism in February, describes a self-regulation mechanism that stops the immune system from overreacting to malaria. By doing so, the immune system prevents damage to the human body, but leads to increased multiplication of the protozoa, thus worsening the disease.
A team led by Ricardo Gazzinelli, an immunologist from the Federal University of Minas Gerais (UFMG) and the Oswaldo Cruz Foundation (FIOCRUZ), conducted animal experiments that simulated human malaria. The scientists found that the second phase of the immune response, in which the body prepares to act against the invader with full force, does not occur as expected. In malaria patients, a chemical signal that induces the maturation of dendritic cells, which are responsible for amplifying the immune response by recruiting more immune components against the invader, causes the opposite to the desired effect. Instead of preparing dendritic cells to recruit other cells that act specifically against the parasite, the signal disorganizes the way the dendritic cells function and slows down the immune response. The result? The protozoan has more time to reproduce undisturbed, intensifying the symptoms of the disease.
“This alteration in the activity of dendritic cells is a form of immunological self-regulation,” explains Gazzinelli, who is also a professor at the University of Massachusetts, USA, where some of the experiments were carried out with funding from FAPESP. “It prevents hyperactivation of the immune system, which can be harmful to the individual. This protects the host organism, but favors multiplication of the parasite.”
Just like infections caused by viruses or bacteria, in people with malaria, the immune response is signaled by a protein called interferon-gamma, a chemical messenger produced by certain immune cells exposed to the invader at the beginning of the infection. Released by natural killer cells and T lymphocytes that had contact with the parasite in the first phase of the immune response, interferon-gamma triggers the next phase of the fight in the spleen. In this fist-sized organ, which plays an important role in the immune system and is located in the upper left of the abdomen, the signal stimulates resting immune cells (monocytes) to transform into dendritic cells, which eventually envelop the parasite, break it apart, and present the fragments to another group of lymphocytes called CD8+ T cells. These, in turn, trigger other components of the immune system capable of destroying infected cells. This is what happens when everything goes as expected.
But something different seems to happen with malaria, according to biologist Theresa Ramalho. During a postdoctoral fellowship under Gazzinelli’s supervision in Massachusetts, she infected mice with Plasmodium chabaudi, a species that causes malaria in rodents, and studied the behavior of dendritic cells in their spleens.
Ramalho noted that interferon-gamma disorganizes the functioning of these cells. Initially, the signal induces a predictable and even desirable response in cells that perform intense activities: an increase in the consumption of glucose, the main source of energy.
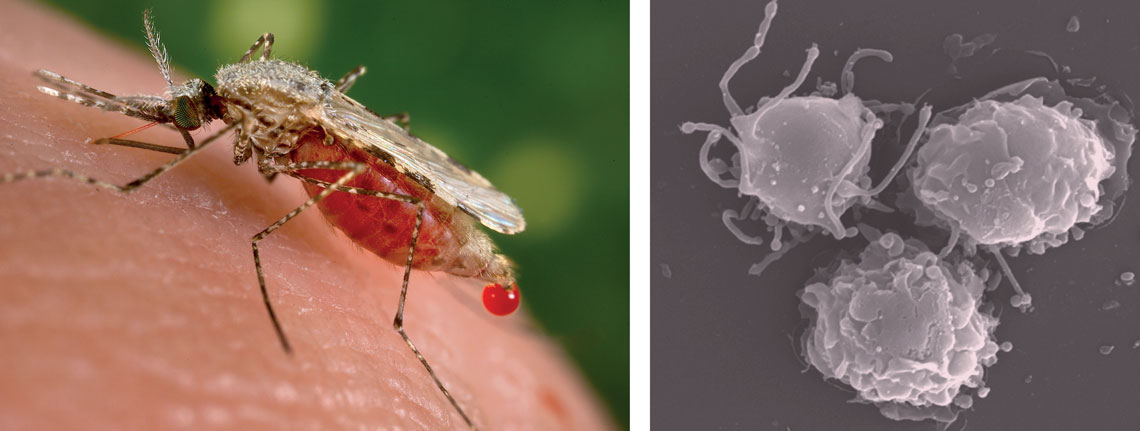
Female Anopheles stephensi, one of the transmitters of malaria, and dendritic cells, the function of which is altered by the disease, seen under an electron microscopeJim Athany / CDC | Ricardo Gazzinelli’s Laboratory / UFMG
The problem is that in malaria, the cycle of transforming glucose into energy is not completed. In the dendritic cells, the interferon-gamma stimulates the production of a protein that stops the process midway. This protein—an enzyme called aconitate dehydrogenase1 (ACOD1)—prevents glucose digestion from being completed and transforms the partially digested sugar into itaconate, a compound that accumulates in the cells and prevents the functioning of the mitochondria, known as the powerhouses of the cells. “In the dendritic cells of infected rodents, itaconate levels were 35 times higher than normal. In the blood, there was four times more,” says Ramalho, lead author of the study.
While it can prevent the growth of bacteria, itaconate does not have the same effect against protozoa, which are also single-cell organisms but are more complex. The increased level of itaconate damages the mitochondria and triggers a sequence of phenomena in the dendritic cells that prevent them from activating CD8+ T cells. “The parasite takes advantage of this situation and multiplies further,” explains the biologist.
Experiments involving rodents genetically altered to not produce ACOD1—which thus do not accumulate itaconate—showed that absence of this enzyme restored activation of CD8+ T cells. “Without itaconate, the mice fought off the infection better, and the amount of plasmodium in the blood was lower,” says Ramalho.
The accumulation of itaconate observed in the experimental malaria model appears to occur in human disease too. The researchers found that in people infected with Plasmodium falciparum, which is predominant on the African continent and causes the most lethal form of malaria, dendritic cells produced more ACOD1. Similar results were seen in infections by Plasmodium vivax, the most common outside Africa, which is generally associated with a milder illness. Itaconate levels were higher in the blood of people infected with this species of the parasite.
Malaria experts not involved in the study called the findings promising, pointing out that they could help in the formulation of new therapies against plasmodium. “Both itaconate and the ACOD1 enzyme are potential targets for clinical interventions,” says FIOCRUZ biologist Cristiana de Brito, who believes itaconate could also be used as a marker of the disease. “Measuring blood levels could allow us to identify people at greater risk of developing severe malaria,” she explains.
Silvia Boscardin, a biomedical scientist from the University of São Paulo (USP), shares the same vision. She highlights that the research offers convincing evidence that itaconate can also play an immunosuppressive role in humans. “This raises the possibility of testing drugs that reduce the accumulation of this compound, when available for clinical use, to assess whether they could improve the clinical condition of patients,” she says.
Project
Immunological mechanisms of malaria resistance and pathogenesis (nº 16/23618-8); Grant Mechanism Thematic Project; Principal Investigator João Santana da Silva (USP-RP); Investment R$7,350,121.64.
Scientific article
RAMALHO, T. et al. Itaconate impairs immune control of Plasmodium by enhancing mtDNA-mediated PD-L1 expression in monocyte-derived dendritic cells. Cell Metabolism. Feb. 6, 2024.