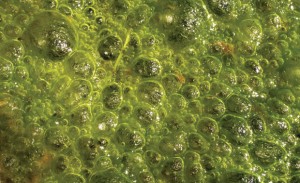
UC SAN DIEGOAlgae: betting on synthetic biology to produce fuelUC SAN DIEGO
Imagine if, based on a complete sequence of your genome, you, the reader, could synthesize your own DNA, introduce it into a human cell and then cause this DNA to take over the command of this cell, forming tissues, organs and even an identical copy of yourself to whom you could transfer your memories.
As in Phillip K. Dick’s science fiction novel that gave rise to the script of the movie Blade Runner, it seems that at least one important proof of the viability of this concept has been achieved. On May 20, the J. Craig Venter Institute (JCVI) published on the website of the journal Science an article in which it reports the activation of the synthetic genome of one microorganism into another one, the bacterium Mycoplasma mycoides. The measure of success, in this case, was that the synthetic genome acquired control of another cell and that this cell began reproducing in a laboratory.
Founder of JCVI, scientist Craig Venter has said in several interviews that he will now go after the genome of an alga to produce bioenergy. I heard him say something similar at a talk delivered at the last World Congress of Biotechnology held in Barcelona in 2009. One of the major challenges we currently face is how to produce bioenergy cheaply and sustainably in environmental terms. The JCVI discovery opened up a path for researchers to obtain engineered microorganisms that do the work of producing ethanol or biodiesel to an excellent standard.
Here in Brazil, at CTBE, the National Bioethanol Science and Technology Laboratory in Campinas, we are already engineering bacteria and fungi with enzymes that attack the vegetable cell wall and that may help in the development of the technological route to second-generation ethanol. One of the laboratories at INCT (National Institute of Bioethanol Science and Technology ), headed by the researcher Richard Ward, reported at INCT’s last workshop, held in April, trials with a chimerical enzyme, i.e., an artificially assembled protein that can attack two components of the cell wall, lignin and hemicellulose, at the same time.
Still, even if we have not achieved anything as spectacular as Venter (yet), Brazilian bioenergy is beginning to enter the synthetic biology era. There are several paths to choose from and problems to solve using this technology. One of them is engineering the metabolism of Saccharomices cerevisiae, the yeast we use to make alcohol, in such a way as to enable it to use the five-carbon sugars that come from hemicellulose, something this yeast cannot do very well.
With microorganisms, the synthetic biology applications will became far faster and should yield impressive results. On the other hand, when it comes to more complex organisms, applications might take rather longer. Nevertheless, biologist are moving in this direction and they are doing it by gaining an understanding of organisms as complex systems.
A major but still initial step
Inserting a synthetic genome into a cell is undoubtedly an important step in the biology area. If one considers that a gene corresponds on average to 10 to 15 kbps (1 kbp equals 1,000 DNA bases), what the JCVI group achieved was building a genome of 600 to 1,000 genes, transferring this to a cell whose DNA had been removed, and making this bacterial cell that became the host of the synthetic genome work.
This is sensational technical progress and its implications are huge. However, the difficulties of applying this to more complex organisms are even greater. This is the case because the genome of a corn plant, for instance, holds an estimated 32 thousand genes. In other words, it is 32 times greater than the synthetic genome that JCVI used. It is likely that in our sugarcane we have roughly the same number of genes as in corn. However, unlike the Mycoplama mycoides, sugarcane has eight copies of each gene. This means that there are nominally some 240 thousand genes interacting in the organism’s genome and causing it to function perfectly well, to the point of allowing us to make the ethanol that we use to fill our gas tanks. If the degree of difficulty were linear in relation to genome size, doing with sugarcane what was done with the bacterium M. mycoides would be 400 times harder. However, there are added difficulties that make this relation even more complex and difficult.
The bioenergy that we need, in part because of our engine technology, is stored in the bonds between carbon atoms and the only way of storing this energy in this way is through photosynthesis. There are bacteria that can carry out photosynthesis and they are generally ranked among the targets of synthetic biology. The cyanobacteria, for example, are good producers of lipids that can function as biodiesel, indicating that we can think about setting up industrial systems to produce bionergy using them.
However, there is an important biological reflection to be considered. If cyanobacteria are that good to produce bioenergy, why is it that to this day civilization has not used them to obtain food and energy? Why is our food based principally on plants that grow on the land? One of the answers is that the increase in complexity that resulted from the evolution of multiple-cell beings and the development of increasingly more efficient photosynthesis systems caused plants to take over the planet. Amongst these, the grass family, such as corn and sugarcane, produced one of the most efficient photosynthesis systems that exist. They have a photosynthesis system called C4, with which they produce a greater amount of biomass in less time than other plants. And this is why civilization such as we know it is heavily based on these species.
Synthetic biology is being adopted to alter photosynthesis in plants. The photosynthesis of soy, for instance, is not as efficient as that of the grass family. However, an international research group has been outlining strategies for implanting, with the help of synthetic biology, a C4 system in the leaves of this legume. This is an immensely more difficult target than JCVI achieved, because we are not dealing only with one genome but, in the case of the C4 system, with three different genomes: one that is in the cell’s nucleus and another two that are found in the two types of chloroplasts in the different cells of the leaves of C4 plants.
Venter took a major step, but a lot of investigation and creativity will still be required for us to really break the code of complexity concealed within life. There is a large number of researchers, even in Brazil, who are turning toward the use of synthetic biology as the main tool for the development of new biotechnologies. The future promises to be great fun and interesting.
Marcos Buckeridge is one of the coordinators of the Bioen-FAPESP program and the scientific director of CTBE, the National Bioethanol Science and Technology Laboratory
Republish