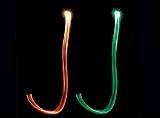
eduardo cesarEntangled photons: shared propertieseduardo cesar
In a space millions of times smaller than the point of a needle, nature behaves very curiously. In this ultramicroscopic world, two or more particles – or even two or more distinct sets of particles – can share a peculiar property that challenges intuition and sometimes is reminiscent of telepathy. Forecast in 1935 and proved experimentally in the 1960’s, this special property called quantum entanglement is a sort of sealed pact that the particles or sets of particles maintain to comply with a given condition. Without anything corresponding in the macroscopic world – governed by the laws of physics proposed by Isaac Newton, which explain, for example, the fall of a ripe mango from the tree down to the ground -, this property seems obscure even to the physicists, who have not yet quite understood it. But the support of an analogy helps to understand this phenomenon, the details of which are now beginning to be known, with the contribution from physicists from the State University of Campinas (Unicamp).
It is easier to explain the entanglement when the particles are left aside and, and, as physicist Daniel Turolla Vanzella, from the University of São Paulo usually does, one thinks of a pair of dice – the ones with six faces, used in board games. First you have to make them entangled. So imagine a machine for entangling dice. With the dice inside this fictitious device, the next step is to choose the characteristic that the dice ought to share – let’s say that the faces turned upwards always add up to 7, after the dice are thrown. Once the program is keyed in, the handle is turned and… voilá – a pair of entangled dice.
In a test, the first die is rolled on the table, and the upper face shows 2. The agreed entanglement guarantees that, when cast, the second die can only give as a result the surface with number 5. If once again one of them is thrown and stops with the No. 6 face on top, the other will certainly show number 1. Odder still: before throwing either of the dice, it is not possible to know which the number will be that each of them will show individually. Only after one of the dice is cast does the other become obliged to show the face that, added to the previous one, gives 7 as the result.
Those who are not convinced that particles can behave this way are not an exception. Physicist Albert Einstein, who foresaw this phenomenon in 1935, in a study with Boris Podolsky and Nathan Rosen, also looked on entanglement with misgivings. Although it is impossible for the dice on a board – just like all other macroscopic objects – to show this behavior in reality, the experiments carried out in the laboratory confirm that it is just like this that nature behaves in the world of the particles, in which distances are measured in nanometers (millionths of a millimeter), explains physicist Antonio de Toledo Piza, from USP.
In two theoretical studies published at the beginning of this year, physicists Carlos Escobar and Daniel Rigolin, from Unicamp, help to reveal some subtleties of quantum entanglement. In general terms, they found that the intensity of the entanglement between two components of a system – whether they are two distinct particles, or two sets of particles – diminishes as the distance increases between one component and the other. Back to the dice, it is as if the entanglement were to weaken as one die is taken far away from the other, reducing the certainty that the second item will stay with 5 on the upper face as soon as the first reveals a number 2.
Another discovery is that the intensity or degree of entanglement between components with different energy levels is lower than between components with similar energy levels. Perhaps another comparison would be helpful. The trustworthiness of the pact between the dice being fulfilled is lower when one of then is shaken more energetically – and the other, less forcefully – than when both are shaken with the same intensity. Accordingly, the certainly that the dice will show the programmed result – a sum of 7 – is greater when both are shaken very strongly, or with lesser intensity, provided that the same level of vigor is maintained.
In a physics laboratory, of course, there are no researchers throwing dice on a table. Instead of this, they work with different sets of particles with a negative electrical charge (electrons), which move around the nucleuses of the atoms, or even with laser beams, sets of billions of particles of light, or photons. The behavior of the particles, though, is no different from that of the dice endowed with this almost magic property.Complicated? Perhaps so. But it is this physical property that makes viable the development of futurist technologies like quantum teletransportation, as the transfer of information proper to one particle to another distant one is called – nothing to do, at least for the time being, with the comfortable form of traveling of the Star War series, because the particle is not transmitted in the process, only its characteristics.
It is also believed that this property makes possible the commercial production of ultrasecure systems for encoding information, quantum cryptography. The present-day cryptography systems, like those used by the banks, are based on the entanglement of the transmitted data, which can only be read by those who know the numerical code, a number with thousands of digits that gives access to the correct information. Quantum cryptography takes advantage of properties of the particles to encode the information. Accordingly, the message become unbreakable, since the subtlest attempt at espionage alters the information – as guarantees the Uncertainty Principle, formulated by physicist Werner Heisenberg – and denounces the pilferer.
Little by little, theory is reaching practice, albeit in an experimental form. The first physicist to demonstrate quantum teletransportation, in 1997, the Austrian Anton Zeilinger, from the University of Vienna, in April achieved another notable feat: he used entangled photons to encode information during the transfer of a donation of 3,000 (about R$ 12,000) from the city hall of Vienna to the laboratory’s account with the Austria Creditanstalt bank, instead of the bank’s usual system of cryptography.
The two Brazilian studies are contributing towards an understanding of this phenomenon, by showing how quantum entanglement should behave in conditions closer to the real ones. Published in Physical Review A, the works gained prominence in two virtual reference publications in this area, the Virtual Journal of Quantum Information and the Virtual Journal of Nanoscale Science and Technology. In the article of January 13, Escobar and his pupil for a doctorate Gustavo Rigolin assessed the intensity of the entanglement between sets of particles with a different energy patterns, for example, laser beams of different colors, like red and blue – the colors are determined by the level of energy with which the photons vibrate, like the dice shaken with different intensities.
Because of the difficulty of the calculations, the exact values of the degree of entanglement were not reached in this case. But Escobar and Rigolin presented two different ways of calculating the minimum limit from which one can consider that these sets of particles are entangled. In this study, they also found that the level of entanglement between sets of particles with different levels of energy – or, as physicists say, are to be found in non-symmetrical states – is lower than that attained by sets of particles with the same level of energy (symmetrical states).
In another way, the physicists have helped to determine the minimum intensity of this connection, as diaphanous as it is intimate, classified by Einstein himself, almost 70 years ago, like a phantasmagoric action at a distance. It is a considerable advance in relation to that achieved by the team of Reinhard Werner, from the Technical University at Braunschweig, Germany, which in 2003 determined the degree of entanglement for sets of particles in symmetrical states, like two laser beams of the same color.
The results of the team from Campinas are important for meaning a step in the direction of what happens in reality. In a way that is different from what happens in the theoretical forecasts – which take into account ideal conditions -, in practice, there are interferences of all kinds, and even the quality of the optical fiber can alter the energy pattern of the laser that carries them. For this reason, knowing the limit from which sets of particles in non-symmetrical states share this property is essential for creating truly efficient systems for cryptography, teletransportation or quantum computing. Furthermore, the more entangled are the sets of particles, the more useful they are from the technological point of view, since the degree of entanglement is directly related to the facility for manipulating the information – for example, for calculations on a future quantum computer – or to the probability of making it arrive intact at its destination in quantum teletransportation, Rigolin explains.
In the second article, published on April 9, Escobar, Rigolin and Lea Ferreira dos Santos, today a researcher at the Michigan State University, United States, analyzed how the degree of entanglement varies in a system that simulates a quantum processor. Instead of photons, the physicists adopted as a model for controlling the characteristics of electrons by means of the alteration of the magnetic field. In a single file of electrons arranged side by side at equal distances from one to the other, like the beads of a stretched necklace, the degree of entanglement diminishes as the distance increases.
Perhaps it is easier to think of a series of snooker balls laid out along a line in this order: white, brown, green, yellow, blue, pink, red, and black. The team from Unicamp saw that the intensity of this interaction between the electron in the position of the white ball and the one in the position of the brown one can attain 80% of the maximum possible degree of entanglement, while this level works out at around 60% between the electron in the place of the white ball and the one in the position of the green ball – and so on. In this same work, an unexpected result emerged. The physicists found that, in some cases, the intensity of the entanglement reaches its lowest degrees when the magnetic field close to each electron varies in a random manner (a chaotic regime) than in the situation in which the magnetic fields are homogeneous – precisely the contrary to what earlier studies indicated.
Furthermore, the maximum levels of entanglement arose at the transition between the chaotic regime and the homogeneous (non-chaotic) regime. It is fundamental detailing for determining how reliable a real quantum processor would be, built according to this model on a semiconductor material – like electrons imprisoned in quantum points (pyramids with a few nanometers in height) of indium arsenide deposited on gallium arsenide. “It would be ideal to keep the magnetic field homogeneous, but in systems like this one, any imperfection in the material makes the regime chaotic”, says Rigolin.
Republish