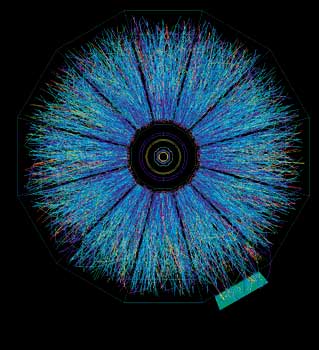
RHIC/BNLIn the detector: particles leave post-collision traces RHIC/BNL
The atomic nuclei of the chemical element gold are made to collide at 99.995% of the speed of light, with enough violence to produce temperatures hundreds of thousands of times higher than those of the external layer of the Sun in a region of space far smaller than the tip of a needle. It is one of the most extreme situations that physicists can create within a laboratory and it is similar to what must have existed fractions of seconds after the Big Bang, the explosion that gave rise to the Universe some 13.7 billion years ago. Although they can reproduce such energy conditions, physicists do not understand very well why the particles that result from this collision spread the way their equipment shows. Or, better said, in the past they did not understand it. However, in the January 25 issue of Physical Review Letters, an article by an international group that included one researcher from CBPF, the Brazilian Center for Physics Research in Rio de Janeiro, started undoing the mystery.
Driven by speeds close to that of light, 79 positively charged particles (protons) and 79 neutral particles (neutrons) of the nucleus of gold reached such a high level of energy that this caused them to break down into even smaller and more elementary particles: quarks and gluons. Previously entrapped, the quarks and gluons started moving freely within a cloud of particles that physicists call plasma, the fourth state of matter (the other three are solid, liquid or gaseous). When two clouds traveling in opposite directions meet, quarks and gluons collide and annihilate each other, after which they regenerate.
Like a phoenix
In this process of subatomic death and rebirth, however, the number of annihilated elementary particles is not always equal to the number of particles created immediately afterwards. Often the collision of a quark with a gluon, of a quark with another quark or of a gluon with a gluon results in three elementary particles rather than two. As the newborn elementary particles pull away from each other after the collision, the plasma cools down and the free quarks and gluons join again, forming larger particles, such as protons and neutrons. As the equipment does not detect quarks or gluons, indirect indications of what took place within the plasma are what the physicists see.
In the experiments conducted in the Relativistic Heavy Ion Collider (Rhic), which is the particle accelerator at the Brookhaven National Laboratory in the United States, physicists used to witness results that were different from their expectations. When they caused heavy nuclei, such as those of gold, to collide against each other, they detected indications of the generation of three elementary particles within the plasma more often than the theory predicted – and more often that what was observed in the collisions of hydrogen nuclei, which are lighter and only comprise one proton.
Partnering physicists from Mexico and from the USA, Javier Magnin, from CBPF, analyzed these results and reached a fairly plausible explanation. “The likelihood of generating two or three elementary particles is the same for heavy nuclei or light nuclei collisions,” says Magnin. “But in the gold collisions we find signs of the production of three particles more often for reasons of geometry,” he explains.
The reason is simpler than one might imagine and is connected with the path of elementary particles within the plasma. In the events in which three elementary particles are generated, one of them always covers a smaller distance than that of those that result from the collisions that generate two particules until escaping from the plasma and generating a detectable particle.
This type of experiment, according to physicists, may help us understand what happened soon after the Big Bang, when an ultrahot and dense plasma of quarks and gluons comprised the entire Universe. “We still don’t know, for example, how plasma evolves and whether it turns into a particle gas,” comments Magnin.
Republish